Summary
- More than 2,000 species of coral have been identified and described.
- Most coral reefs are found in the tropics and subtropics.
- More than 200 coral species are listed as threatened with extinction on the IUCN Red List. Although recent research suggests this figure could be an overestimate.
- Corals face multiple threats, including mass bleaching, overfishing, pollution of local waters, and ocean acidification.
- Mass bleaching events are becoming more common and severe.
- The time between bleaching events is getting shorter – often too short for corals to recover from.
- Large bleaching events used to be reserved for warm El Niño years. Now they even occur in La Niña years, the ‘cold’ phase of the ENSO cycle.
- Some corals die immediately when exposed to warming. Others bleach, then either recover or die.
- Some corals are much more resilient to warming than others.
- It’s unclear how corals will respond to ocean acidification over the next century.
- More experiments of coral responses at lower levels of warming and acidification are needed.
How many coral species are there?
Our oceans are home to many types of coral – some shallow, warm water species; others cold, deep-water species. But just how many species of coral are there?
As with any other group of wildlife, it’s hard to say with complete certainty: this is because there is always the possibility that there are coral species that we’re yet to discover. New animal species are unearthed every year.
What we can say is how many species have been identified, named and described. These are listed by the IUCN Red List and updated every year.
In the chart we see the number of described species across a range of taxonomic groups. Due to the issue of finding and counting every species, these are likely to be underestimates.
We see that there are more than 2,000 described coral species in the world.
Coral reefs across the world
Coral reefs are some of the most beautiful and diverse lifeforms on the planet. Yet they’re also some of the most misunderstood. Are corals animals, plants, or something else? What happens when a coral dies; can it come back to life?
The fact that they live in our oceans means that, like much of the planet’s marine life, only a tiny fraction of people will ever see one up close. Yet they provide immense value to communities across the world. More than 450 million people across more than 100 countries live close to coral reefs and rely on them for their livelihoods.1 They generate an estimated $375 billion per year through the goods and services they provide.2
They’re so valuable because they form the bedrock of diverse ecosystems built on top of them. You will only find corals in small areas of the world’s oceans – they cover only 0.5% of the ocean floor. Yet they support almost 30% of the world’s marine fish species.3 Reefs in the Indo-Pacific region alone (spanning Australia and Southeast Asia) are home to more than half a trillion corals.4 That’s more than the number of trees in the Amazon rainforest.
Corals are animals that belong to the phylum of cnidarians – a group of more than 11,000 water-based animals. Most live in marine environments. There are two main types of corals: shallow, warm-water corals; and cold, deep-water corals. The obvious difference between the two is that warm-water corals live close to the sea surface – usually in coastal waters – whereas cold-water reefs can extend to depths of 3,000 meters below the surface.5
Warm and cold-water reefs therefore function in very different ways. Corals use calcium carbonate in ocean waters to build a hard exoskeleton. But the key to their success is how they get their energy. Warm-water corals contain microscopic algae called zooxanthellae in a symbiotic relationship. The algae photosynthesize for them, providing corals with most of their energy.6 These corals wouldn’t be able to survive without them. They can only do this by living close to the surface where light is abundant.
Once we reach 200 meters below the surface – which is where most cold-water corals live – there is so little light that photosynthesis is not possible. Cold-water corals don’t have these symbiotic algae, and get their energy from feeding on particles on the ocean floor instead.
A reef is then an assemblage of corals. Much like a collection of them. Reefs can be composed of many different types of coral – there are more than 2,000 identified species. Each can form unique structures and grow at very different rates. Acropora palmata, for example, is a reef-building coral found in the Caribbean. It forms a complex structure with branches that look a bit like antlers, which is why it’s commonly known as ‘Elkhorn coral’. It grows very quickly, at around 5 to 10 centimeters per year. Contrast that with the species, Porites lichen, which are common in lagoons and form very flat and smooth plate-like structures. Usually a shockingly bright yellow. Or so-called ‘brain coral’ – most common in the Favia genus – which form round structures with grooved surfaces much like the human brain.
Reefs can therefore form with a wide array of different species, leading to highly complex structures. Each of these species has its advantages and disadvantages. The branching Acropora palmata, for example, can break more easily when exposed to storm or wave damage. But it grows back very quickly. Brain coral is much more resilient to storms because it’s spherical, compact structure is harder to break down. The downside is that it grows more slowly. These differences matter for how corals respond to increasing pressures, and how the structure of reefs might change over time.
You will find most coral reefs in the tropics and subtropics.
Warm-water corals – which cover around 0.1% of the ocean surface – tend to live within 30° of the equator. Their optimal water temperature is between 23° and 29°C.7 In fact, coral species tend to live in waters at the very top of their temperature optimal range which is bad news for their prospects as the oceans warm. A temperature increase of a degree or two is enough to push them past the threshold they can tolerate.
The distribution of the world’s warm-water coral reefs is shown in the map. Each box represents a gridded location where NASA collected high-resolution imaging and data on the ocean’s corals as part of its Millennium Coral Reef Mapping Project.
There are several key reef clusters across the tropics. We often distinguish them based on their geographical location, or ocean they’re in. Caribbean reefs; the Great Barrier Reef; the Red Sea; Arabian Sea; and Persian Gulf reefs.
The Great Barrier Reef is the most well-known. It’s the largest coral reef system in the world, extending 2,300 kilometers from the Torres Strait, just south of Papua New Guinea down the eastern coast of Australia. In fact, it’s the world’s largest structure built from living organisms. It’s even clearly visible from space.
It, like the many other reef systems, is home to some of the planet’s most diverse habitats. But they’re increasingly threatened from a range of pressures: overfishing, water pollution, coral bleaching and ocean acidification. If we want to protect these ecosystems from decline we need to take action. If we don’t we risk damaging them beyond repair.
How many coral species are threatened with extinction?
Over the course of Earth’s history, corals have seen multiple episodes of extinction. In each of the ‘Big Five’ mass extinctions, there were intense periods of global warming or cooling, and dramatic changes in the chemistry of the world’s oceans. Each time, corals across the world’s oceans went extinct.
Although there have been no recorded coral extinctions in recent centuries, a large number are still threatened with extinction today.
The IUCN Red List of Threatened Species evaluates species across the world for their level of extinction risk. The IUCN has not evaluated all of the world’s known species; in fact, in many taxonomic groups it has assessed only a very small percentage. Only 1% of known insects, for example, have been assessed. The coverage for birds is much better: in fact, 100% of known bird species have been evaluated for their extinction risk.
We should also be clear about what ‘threatened with extinction’ actually means. The IUCN labels a species as threatened if it falls into one of the following three categories.
- Critically endangered species have a probability of extinction higher than 50% in ten years or three generations;
- Endangered species have a greater than 20% probability in 20 years or five generations;
- Vulnerable have a probability greater than 10% over a century.
In the chart we see the number of species threatened with extinction across taxonomic groups. It estimates that over 200 corals species are threatened with extinction.
Emerging research suggests this number could be too high. In a study published in Nature Ecology and Evolution, Andreas Dietzel and colleagues undertook a large-scale assessment to estimate the total number and population size of more than 300 coral species spanning the Pacific Ocean.9 It’s hard to conduct such large-scale assessments of coral populations and so this study fills a large gap in the existing research.
What became clear is just how abundant corals are. There were around half a trillion corals across these reefs – more than the number of trees in the Amazon. Two-thirds of the species had population sizes exceeding 100 million colonies, and one-fifth had populations of more than one billion.
Their findings suggest that while the loss of coral cover and severe local depletions of corals is a very clear and imminent risk, the threat of total extinction of species is likely to be lower than previously suggested. In fact, they found examples where species currently listed as ‘threatened’ were massively abundant. 12 of the 80 species in their analysis that were listed as ‘threatened’ on the IUCN Red List has populations of over one billion colonies. And, conversely, some of the rarest species in their analysis – with much smaller populations – were listed as ‘least concern’ on the Red List.
This research suggests that while local or regional extinctions of corals are possible in the near- or medium-term future, global extinctions of Indo-Pacific corals would take much longer. They suggest that a major update to listed extinction risk of the world’s corals is urgently needed.
Coral reefs are home to some of the most diverse and oldest ecosystems on Earth.
Like all lifeforms they have faced a range of threats over their history, encountering periods of intense pressure and period of relative calm. But humans are putting increasing pressure on these ecosystems in a number of ways. These threats are becoming more frequent and intense than many corals can recover from. If we don’t tackle them then we risk losing many of these beautiful ecosystems in the century ahead.
In this article we look at the threats that coral reefs face today, and how they might respond to these pressures.
What threats do corals face?
When people think of the threats to coral reefs it’s usually bleaching that comes to mind. Bleaching happens when corals expel the algae that they rely on to harvest sunlight – this starves them of their energy source, and can ultimately lead to mortality. This happens when they’re exposed to extreme warming. I look at coral bleaching in more detail here.
But corals face a wide range of pressures. Some of them have naturally occurred throughout history, but many are also human-driven and have accelerated over the last few decades. In the table I have listed the main pressures that corals face today.
This might seem like a long list of problems. But they actually boil down to only a few key drivers.
First is our emissions of greenhouse gases. This can cause three key problems for corals: bleaching from warming surface waters, as we already discussed; an increased risk of damage from storms if climate change makes them more frequent and intense; and ocean acidification from our emissions of carbon dioxide (CO2). Ocean acidification is the result of increased dissolution of CO2 in our oceans which makes them more acidic; this can lead to the dissolution of calcium carbonate which corals use to build their skeletons.
Second is our fishing and shipping activities. Coral reefs are part of an impressive ecosystem of marine life; overfishing leads to the depletion of key species which are needed to maintain these ecosystems. Destructive practices such as fishing with explosives; dredging; boat anchors and shipping lanes can severely damage the physical structure of coral reefs.
Third is pollution and disease. Human development on coastal areas can create large impacts for the chemistry of coastal waters. Fertilizer and manure from nearby farms can runoff into the ocean; coastal populations might have sewage disposal pipes; and developments along the shoreline can cause sedimentation and cloudy coastal waters. This pours excessive nutrients, and potentially heavy metals and toxins into the water. Algae and other microbial lifeforms thrive on these nutrients and can take over the whole ecosystem. They block out sunlight and starve the waters of oxygen. Corals, and the ecosystems they have built around them, can quickly die off.
These are large threats that could transform the world’s coral reefs beyond recognition. But by aggregating them into three key areas we can also focus on three core solutions. Admittedly these are simple in principle, but difficult in practice. First, reduce greenhouse gas emissions. Second, create marine protected areas around coral reefs to limit fishing and shipping activities. Third, regulate coastal development activities around key reef zones.
Type of disturbance | Human pressure | Impact on corals | Solution |
Greenhouse gas emissions | Warmer waters from climate change | Bleaching, resulting in coral mortality | Reduce greenhouse gas emissions |
Ocean acidification from more CO2 | Dissolution of corals, and reduced growth rates | Reduce CO2 emissions | |
Potential increase in intensity and frequency of storms | Physical damage to the structure of coral reefs; in-tact coral can be destroyed | Reduce greenhouse gas emissions | |
Fishing and shipping | Overfishing | Loss of coral and the ecosystems they support | Marine protected areas (MPAs) |
Destructive fishing with explosives | Localised, acute loss of coral reefs | Marine protected areas (MPAs) | |
Shipping lanes, anchors and boat groundings | Destruction of physical structure of coral reefs | Marine protected areas (MPAs) | |
Pollution | Sewage and nutrient runoff from land | Replacement of coral with algae. Higher risk of disease. Impaired growth from toxins or heavy metals. | Marine protected areas (MPAs). Regulation on sewage disposal and coastal agriculture. |
Sedimentation from coastal development | Particles cloud the water and block sunlight, reducing coral growth | Regulations on coastal development around reef zones | |
Invasive species | Possible introduction of disease and competition with existing species | Limit introductions to native species, or carefully selected new species |
Coral reefs have always encountered threats; humans just increasing their intensity and frequency
The threats to global corals – warming waters, ocean acidification, changes in ocean chemistry, and the dynamics of ecosystems – are nothing new. Corals have experienced pressures to varying degrees throughout Earth’s history.
In the very distant past some of these changes were extreme. There have been five mass extinctions in our planet’s history – the so-called ‘Big Five’. Each of them involved large changes in the global climate and the chemistry of the world’s oceans.10 These events were devastating for the world’s corals. After every one there were no living reefs for several million years; this left so-called ‘reef gaps’ in the geological record where there are no remains of living reefs.11
Of course that’s taking the extreme case of extinction events. But even in a present world without humans, corals would still experience some stresses. They would be hit by storms and cyclones. They would experience bleaching events, particularly in warm El Niño years. And they would see period changes in the dynamics of their ecosystems. Corals are put under pressure and they can usually recover over the following years or decades.
What’s changed in the more recent past is that human pressures are increasing the frequency at which these events occur. Rather than bleaching events being reserved for warm El Niño years, they’re happening every year, even in the ‘cool’ La Niña phase. This means they have almost no time to recover before being hit with another mass bleaching event. They can be hit with cyclones more often, or at a higher intensity. And the pressure of overfishing, algal blooms and the depletion of the ecosystems that corals thrive alongside only adds to these stresses.
The dating and extent of these increased pressures varies a lot from region to region. While coral bleaching impacts have only really ramped up since the 1990s [see our related article on the extent of coral bleaching], other pressures date back further.
See, for example, the decline of the primary coral species Acropora in the Caribbean.12 The share of Caribbean reefs where Acropora was dominant or present is shown in the chart. Katie Cramer and colleagues reconstructed these long-term trends in corals and estimated that coral cover in 1950 was similar to what it was 10,000 or even 100,000 years ago. But, from 1950 we see a steep decline: in 1950 it was present at 92% of Caribbean reefs; by the 2000s this had fallen to 19%. What we see is that this decline started well before the onset of more intense bleaching in the 1980s or 1990s. By the 1950s, populations were becoming increasingly developed close to the coast. With these populations came agriculture and increasing amounts of fertilizer. Excessive or poor management of this fertilizer can runoff into coastal waters, creating algal blooms and large shifts in the composition of the marine ecosystem.
This decline is not just a transition from Acropora corals to other species. In a study published in Science, researchers estimate that total coral cover in the Caribbean declined from 50% in 1977 to just 10% by 2001.13
Timing and extent of coral degradation varies from region to region
This degradation of coral ecosystems that we saw in the Caribbean is reflected in other regions of the world, but often not to the same extent. Caribbean reefs have seen the greatest decline over the last 50 years, and have fundamentally changed from ecosystems of coral to ones dominated by algae.
This wider point about the degradation of reef systems is an important one. We often rely on metrics such as coral cover or coral mortality as a marker of reef health. But this does not always give us an accurate comparison of reef health. As we saw discussed earlier, corals have always gone through periods of coral decline and recovery. Simply having low coral cover does not necessarily indicate poor reef health if it is on the path to recovery.14 A reef with 20% coral cover that is restoring quickly is arguably in a better state than a reef with 60% cover that is seeing year-on-year declines.
Many researchers have stressed that it’s not necessarily the absolute level of coral cover that matters for reef health, but where the reef lies between two states of equilibrium. Corals are often described in terms of a phase-shift. In one phase, a reef is resilient to pressures such as bleaching, storms, or disease; when these events occur they feel the short-term impacts but can quickly recover. This is the ‘coral-dominated state’.
But there is another phase: one triggered by a non-linear response. Reefs can be pushed too far and ‘flip’ into an ‘algae-dominated’ state. This is a state where the ecosystems have fundamentally changed from one dominated by coral to one of macroalgae. This new state of equilibrium is difficult to recover from. A reef in this state is an unhealthy one.
To go back to our example of Caribbean reefs: we can see them flip from a coral-dominated to algae-dominated state over time, but also compare them to other regions. In the chart we see the different reef compositions in the Caribbean, Florida Keys and the Great Barrier Reef in the early 2000s.15 We see such a stark contrast between the Caribbean and the Great Barrier Reef. In the Caribbean we find reefs of algae, not of coral: 40% is covered by macroalgae versus only 15% of coral. It hasn’t always been this way: in the 1970s, macroalgae covered only 2% of Caribbean reefs.16 A 20-fold increase. And a phase-shift from coral to algae-dominated reefs. One major shock that contributed to this shift was a disease outbreak in 1983 among the sea urchin, Diadema antillarum.17 This upset the dynamics of the ecosystem and allowed algae to take hold.
Contrast that with the Great Barrier Reef where less than 5% of cover was algae, and 25% was coral. Its reefs are still very much in the ‘coral-dominated’ phase. This means they are resilient to pressures and can bounce back. This is good news: the local protection of the Great Barrier Reef means it has experienced less of the early pressures of fertilizer runoff, sewage and overfishing, but it’s not being hit with more and more mass bleaching episodes [we look at this in more detail here]. To stand a chance of recovering from these events it needs to stay in this coral-dominated, healthy state.
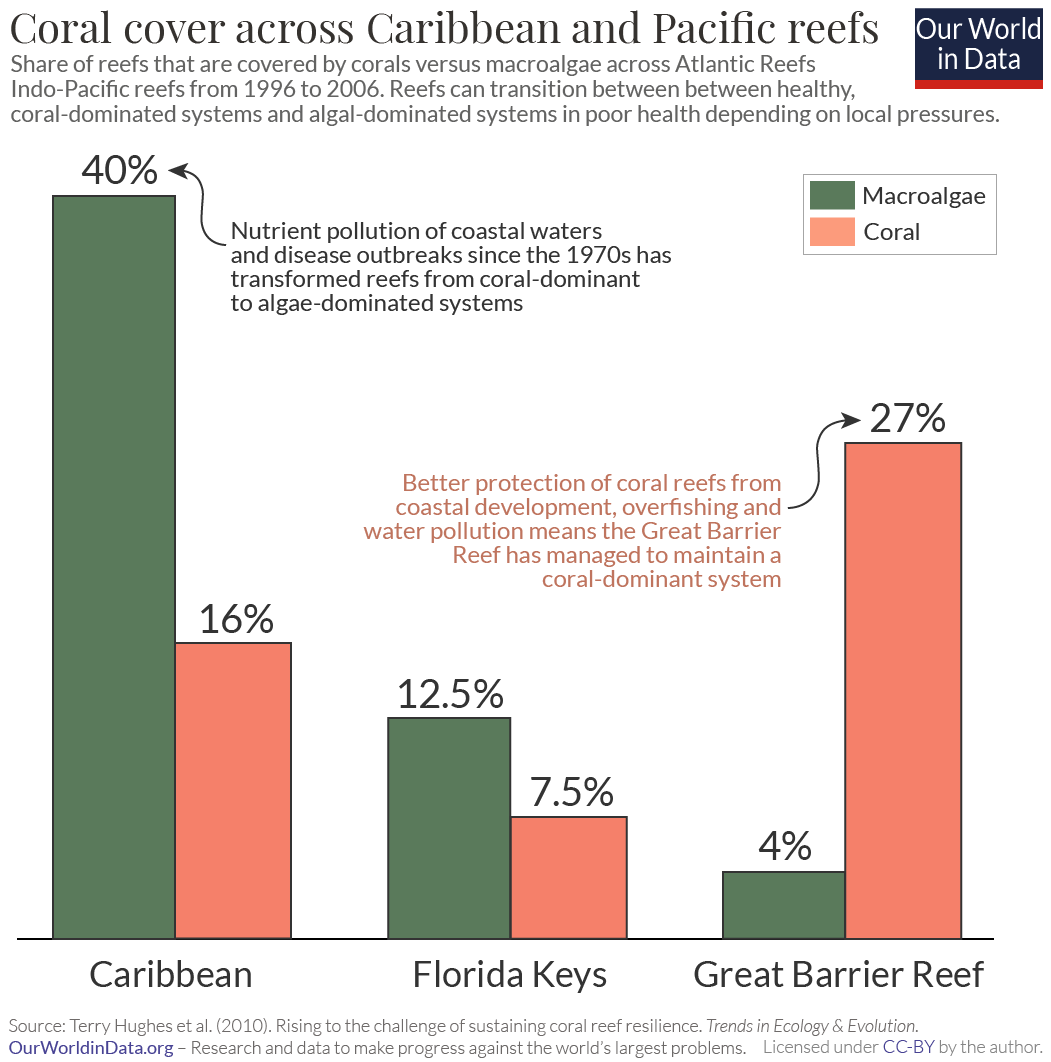
Coral bleaching events are becoming more common and severe
Coral reefs form some of the most diverse ecosystems on the planet. But, for most of us, they are hidden from view. We are blind to how these ecosystems might be changing.
Coral reefs face a number of threats – most of them are increasing with human pressure. One of the most pressing is coral bleaching: potentially fatal events caused by our warming oceans.
In this article, I look at how coral bleaching is changing over time. We see that these events are becoming increasingly common and more severe; they’re now happening more frequently than most corals can recover from.
Corals are animals that belong to the phylum of cnidarians – a group of more than 11,000 water-based animals. Most live in marine environments. Corals use calcium carbonate in waters to build a hard exoskeleton. But the key to their success is their symbiotic relationship with algae. They contain microscopic algae called zooxanthellae that photosynthesize (just like any other plant would), providing corals with the majority of their energy. Corals wouldn’t be able to survive without them.
This important relationship can be disrupted when corals are put under stress. One of the biggest stressors is warmer temperatures. When exposed to warmer waters, corals expel their algal symbionts, meaning they lose their source of energy. Without their algae, corals turn pale; this is why this process is called ‘bleaching’.
Corals do not always die immediately after bleaching. Some do, but most survive. Throughout history, corals have experienced infrequent coral bleaching events.18 They usually recovered over the course of a decade or more. But when corals are hit with bleaching event after bleaching event, in short succession, they struggle to recover. These events often kill them off.
It should seem obvious why coral bleaching is a growing concern for the world’s coral reefs. With climate change, the global average temperature is rising. We often think about atmospheric or terrestrial temperatures, but our oceans are warming too. The global average sea surface temperature has increased by around 1°C since 1850.19 Importantly, the peak temperatures in summer – when bleaching events are most likely to occur – has also increased in recent decades.20
We might expect that corals have been under increasing pressure from warming waters. Do we see evidence for this in the real world?
Many studies have documented an increase in the number of bleaching events across the world’s coral reefs. Satellite data allows us to track specific changes in water temperatures around specific reefs, and the level of thermal stress that they are exposed to. The first study to do this at a global scale found that the percentage of global reefs that were impacted by bleached stress tripled over the 28-year period from 1985 to 2012.21
In a more recent study, published in Science, Terry Hughes and colleagues tracked the frequency of coral bleaching events across 100 pantropical locations from 1980 to 2016.22 This included all of the key coral hotspots spanning 54 countries, from the West Pacific, to the Atlantic, the Indian Ocean to the Great Barrier Reef in Australasia.
They not only quantified the total number of bleaching events but also their intensity. Bleaching events are differentiated into two categories: moderate bleaching occurs when less than 30% of the corals at a given location are affected; severe bleaching is when 30% or more are. Severe episodes represent events where mass, rather than localized, bleaching has happened.
In the chart, we see the number of events that occurred across these 100 reef locations by year. Moderate events are shown in blue; severe events in red. What we see is a general increase in the number of bleaching episodes over time. In the 1980s and ‘90s, it was rare for there to be more than ten events in any given year. From the millennium onwards, it was rare that there would be less than ten.
We also see large spikes in particular years: 1983, 1987, 1998, 2010, and 2016. Most of these events were triggered by the so-called El Niño climate cycle that leads to unusually warm waters in the Pacific. But, as we’ll now see, severe bleaching events might have been restricted to El Niño years in the past but a warming planet means this is no longer the case.
The El Niño–Southern Oscillation (ENSO) is a natural climate phenomenon that affects surface water temperatures in the eastern Pacific Ocean. But it has an impact on the climate across the tropics and subtropics more broadly. It transitions between the El Niño part of the cycle, which is the ‘warm phase’, and the La Niña which is the ‘cool phase’.
As we might expect, bleaching is usually worse in El Niño years when surface waters become warmer. In fact, that’s exactly what we see in the record from a few decades ago. In the chart we see the number of bleaching events across the 100 locations we looked at previously, colored by the phase of the ENSO cycle at the time. El Niño years are shown in red; La Niña in blue; and moderate years between these transitions in grey.
In the 1980s, most bleaching events were reserved for El Niño years. We see examples of this in the 1983 and 1987 spikes. Bleaching was rare in moderate or La Niña years. This was particularly pronounced when we look at severe bleaching events, which is shown in the next chart. There was almost no severe bleaching in the absence of El Niño.
This is no longer the case. Over these decades we’ve seen more and more events in moderate ENSO years, and even in La Niña years, the ‘cold phase’ of the cycle. This applies to the total number of bleaching events, but also the severe ones. Coral bleaching is no longer a rare El Niño occurrence; it happens every year, regardless.
When we look at sea surface temperature data over these decades, this makes sense. In the chart we see the tropical sea surface temperature anomaly – the change in the sea surface temperature relative to the average from 1961-1990.23 El Niño years are shown as the red markers; La Niña years in blue; and moderate in black.
We see is that the temperature anomaly during moderate or La Niña years in the last decade or two is higher than the temperatures during El Niño years a few decades ago. In other words, the ‘cool phase’ of the cycle is now warmer than the ‘warm phase’ of the cycle a couple of decades ago. This means the temperature threshold for coral bleaching is now surpassed in most years of the ENSO cycle when this was reserved for El Niño years in the past.
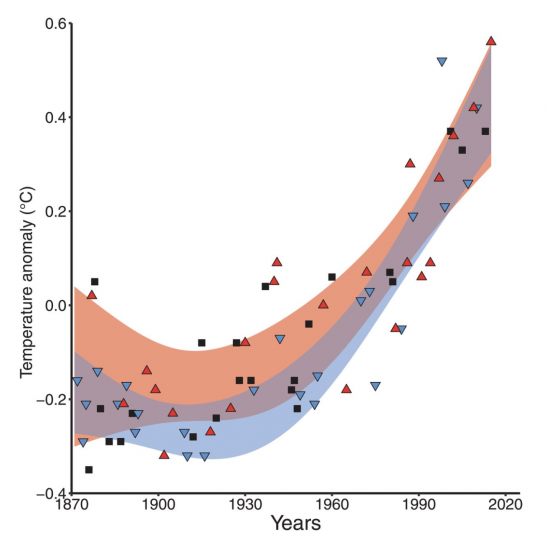
It’s not just the total number of corals that are affected each year that is an issue, but also the frequency with which individual reefs are impacted. As I already mentioned, occasional bleaching episodes have been a constant phenomenon for most corals. Most of them survive. What’s new and concerning is prolonged and repeated bleaching episodes that they don’t have time to recover from.
In the chart we see the frequency of the coral bleaching events across our 100 locations from 1980 onwards. The height of each bar represents the number of locations that experienced the given number of events over these decades. This is shown separately for the total number of events (the pale bars) and severe events (the dark red bars).
By looking at the two extremes of the chart we see just how widespread global bleaching is. On the far left we see that only 3 of the 100 locations did not experience a bleaching event over this period. And, only 9 did not have a severe event. 91% of locations experienced severe, mass bleaching. On the far right we see that many experienced bleaching events every few years. Nearly one-third of locations had eight or more episodes since 1980. That’s one every few years.
Just over half of the reefs experienced two or three severe bleaching events. Some experienced many more. This means the vast majority had a severe bleaching event at least once per decade.
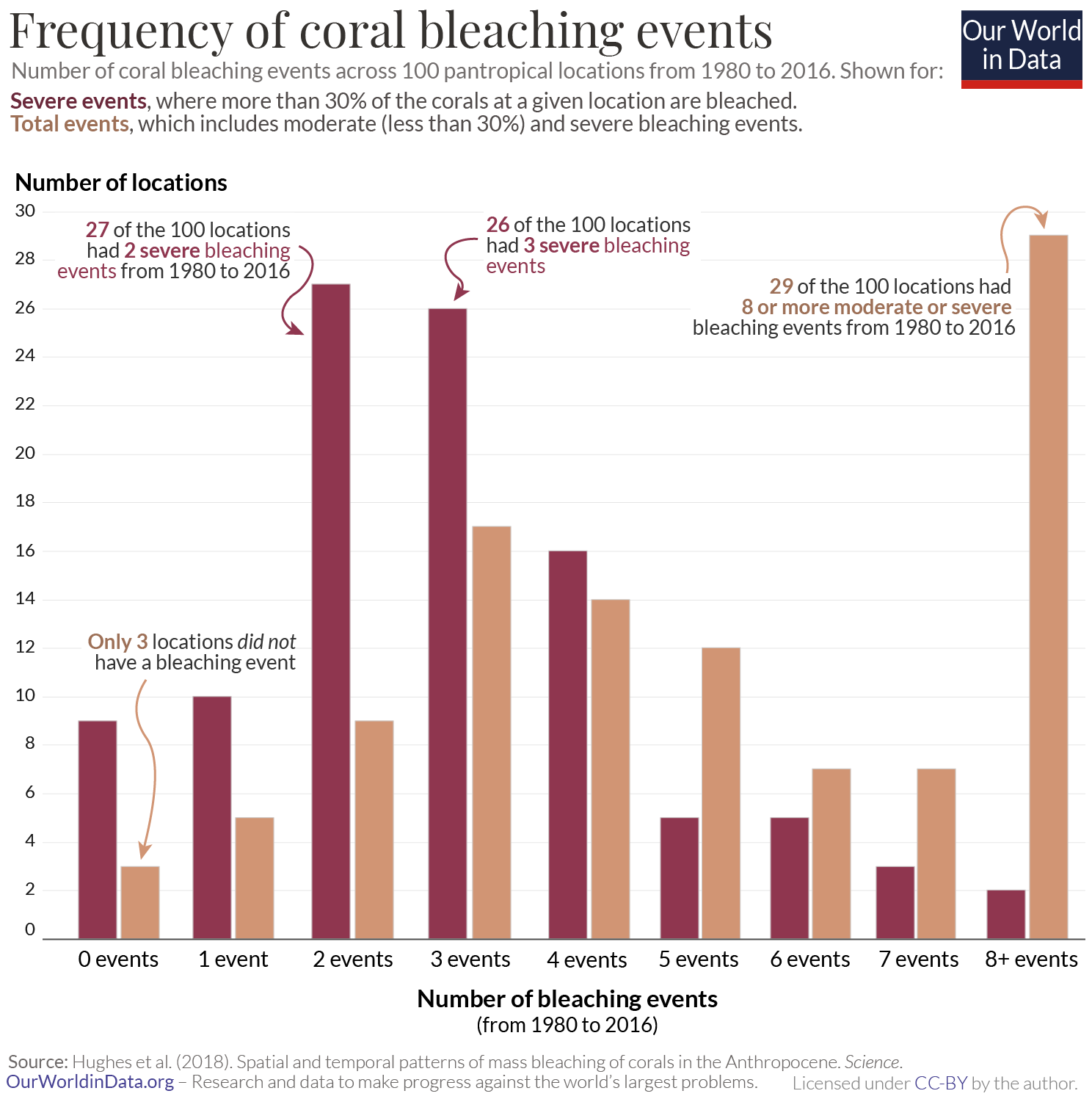
The frequency distribution tells us something about how common bleaching events are. But it doesn’t give us an accurate picture of how the time between events has been changing. Shorter recovery times can mean a higher likelihood of corals dying off.
In the chart here we see the return time of severe coral bleaching events across the 100 locations. This measures the number of years after a severe bleaching event that we would expect another one to hit. In dark blue we have the return times in the 1980s and 90s; in green we have the return times since the year 2000.
Overall, the estimated return time of severe bleaching events has declined from once every 27 years in the 1980s to once every 5.9 years in 2016. That’s a 4.6-fold reduction.
This reduction is visible in the chart. If we look at the blue bars, which show us the return times in the 1980s and 90s, we see a roughly equal distribution: around one-fifth of reefs experienced severe events every 1 to 3 years; one-fifth every 4 to 6 years; all the way to 13 years or more. Contrast that to the green bars, showing this distribution since 2000. It has shifted to the left, towards shorter times. Much fewer locations have return times of ten years or more. And the number of locations with 1 to 3 years; or 4 to 6 years has increased a lot.
What’s concerning is the number of locations that experienced severe events every year or every few years. One-third (33%) of return times since the year 2000 have been just one, two or three years. This is much shorter than the typical 10 to 15 years that most corals need to recover from severe bleaching. Some can recover faster, but most do not.
Shorter and shorter times between events will ultimately lead to the mortality of many corals. It will also change the composition of the reefs that remain. Some species are more resilient than others to warmer waters; these might survive while the less resilient species die.
The ability of coral reefs to adapt to warmer waters has been a hot topic for conservation. In a follow-up article I take a look at the latest research on how adaptable reefs are to warming and what their future prospects might look like.
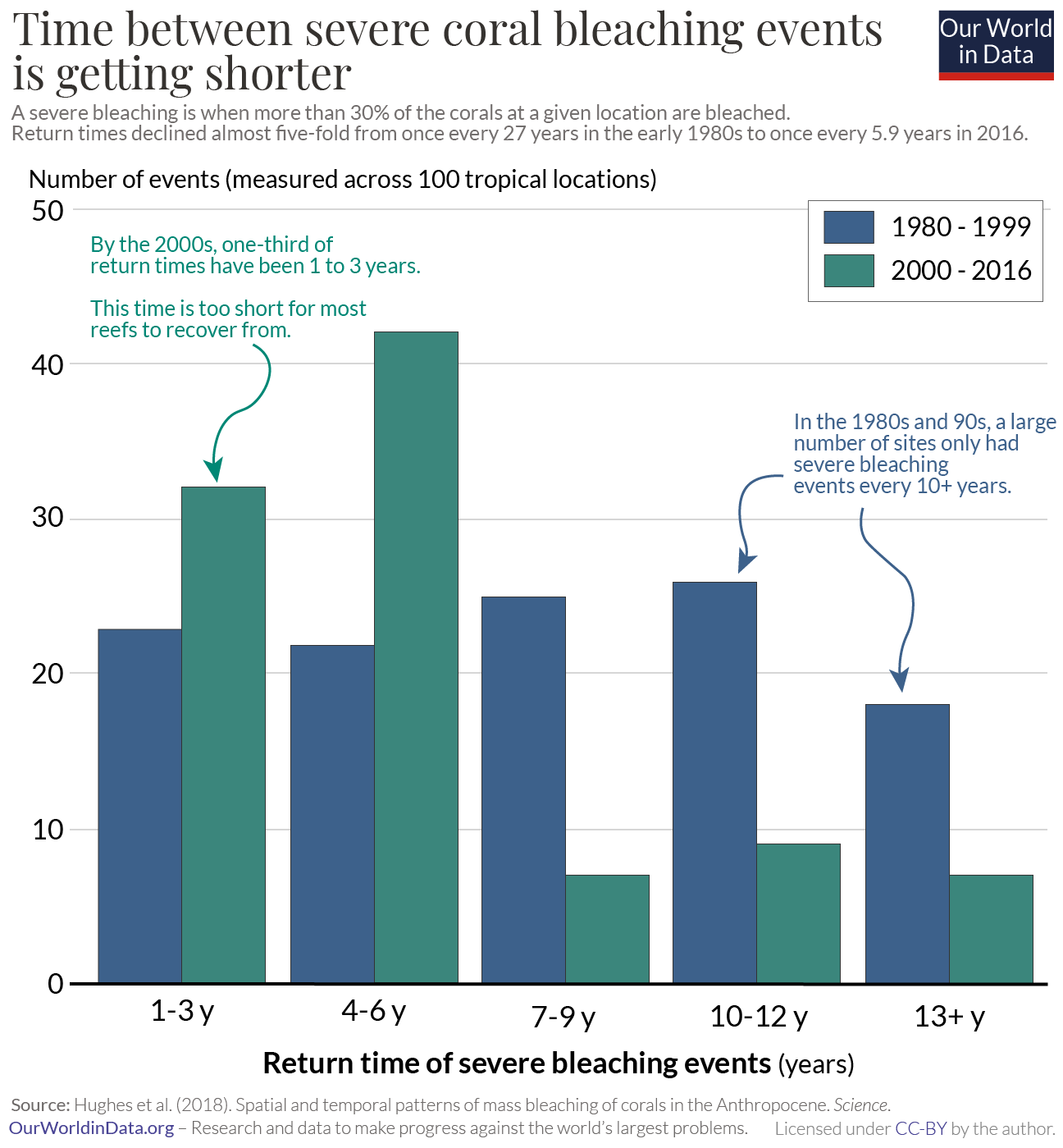
Do coral reefs die when they experience coral bleaching?
Coral bleaching is a phenomenon that many people have heard of, but have never seen in the real world. It’s also not clear to many people what actually happens when coral bleaches. Does it immediately die? Is a bleached coral a dead coral? Can it recover?
The frequency and intensity of coral bleaching events have been increasing as a result of climate change [I looked at the global trends in bleaching events here]. As our oceans continue to warm in the coming decades, we would expect corals to experience even more. We need to understand what happens to a coral when it bleaches, and what its prospects are for recovery if we’re to assess what this means for the future of our reefs.
To do this we’re going to focus on the impact of a single bleaching episode in a specific location. In a study published in Nature, Terry Hughes and colleagues looked at the impact of the record-breaking marine heatwave in 2016 on the Great Barrier Reef – the world’s largest and most well-known coral.24 This gives a detailed look at how corals across a reef ecosystem respond to an extreme period of warming.
We should first briefly cover what coral bleaching actually is.
Corals build a hard exoskeleton using calcium carbonate in ocean waters. But the key to their success is their symbiotic relationship with algae. They contain microscopic algae called zooxanthellae that photosynthesize (just like any other plant would), providing corals with the majority of their energy. Corals wouldn’t be able to survive without them.
When corals are put under stress through extreme temperatures this important relationship breaks down. They expel their algal symbionts and lose their source of energy. Without their algae, corals turn pale; this is why this process is called ‘bleaching’.
Now, let’s turn towards the Great Barrier Reef (GBR) to see how this plays out in reality. Hughes et al. (2018) looked at the impact of warming across 1,156 individual reefs across the GBR ecosystem using high-resolution aerial surveys. They combined this with underwater assessments on 63 reefs spread right along the coast. These reefs covered the full spectrum of heat stress and exposure to bleaching.
They measured a number of key metrics: initial mortality; the extent of coral bleaching; longer-term changes in coral cover; and changes in the composition of these reefs.
All of these metrics were measured relative to the levels of heat stress that corals experienced. This gives us an understanding of what levels of warming need to be reached for corals to die; become bleached; and what stresses they might still be able to recover from. Heat stress is given by a metric called ‘degree-heating weeks’ (DHW). DHW measures the amount of stress that corals have been under in the previous 12-weeks. It’s given as a combination of the length of time that corals are exposed to temperatures above their ‘bleaching threshold’ and the amount that they exceed this temperature by.
A coral could have a DHW value of 2 if:
- local water temperatures were 1°C above the bleaching threshold for two weeks [1°C multiplied by 2 weeks];
- or if local water temperatures were 2°C above the threshold for one week [2°C multiplied by 1 week].
The higher the value of DHW, the more heat stress a reef is under. A large amount of reefs across the Great Barrier Reef were exposed to high levels of heat stress: one-third experienced at least 4°C-weeks and one-fifth experienced 8°C-weeks or more.
Many millions of corals died almost immediately in the northern Great Barrier Reef in March 2016. This happened at the peak of the extreme temperatures over the course of only two to three weeks. This initial mortality was not caused by the normal process of bleaching where corals lose their algal symbionts and ultimately starve. Instead, temperature-sensitive corals died immediately from being exposed to stress that they had no hopes of recovering from.
In the chart we see the percentage of corals on each of the 63 sampled reefs that died off immediately. This is plotted against levels of heat stress, where further to the right means more heat stress. When heat stress was low very few corals died off immediately: fewer than 5% of corals died off on reefs exposed to less than 4°C-weeks. But, as we see, there was a significant drop-off on reefs that were exposed to more heat stress: on reefs with 4 to 8°C-weeks of exposure, the median loss was 16%. For those with more than 8°C-weeks, this was 27%.
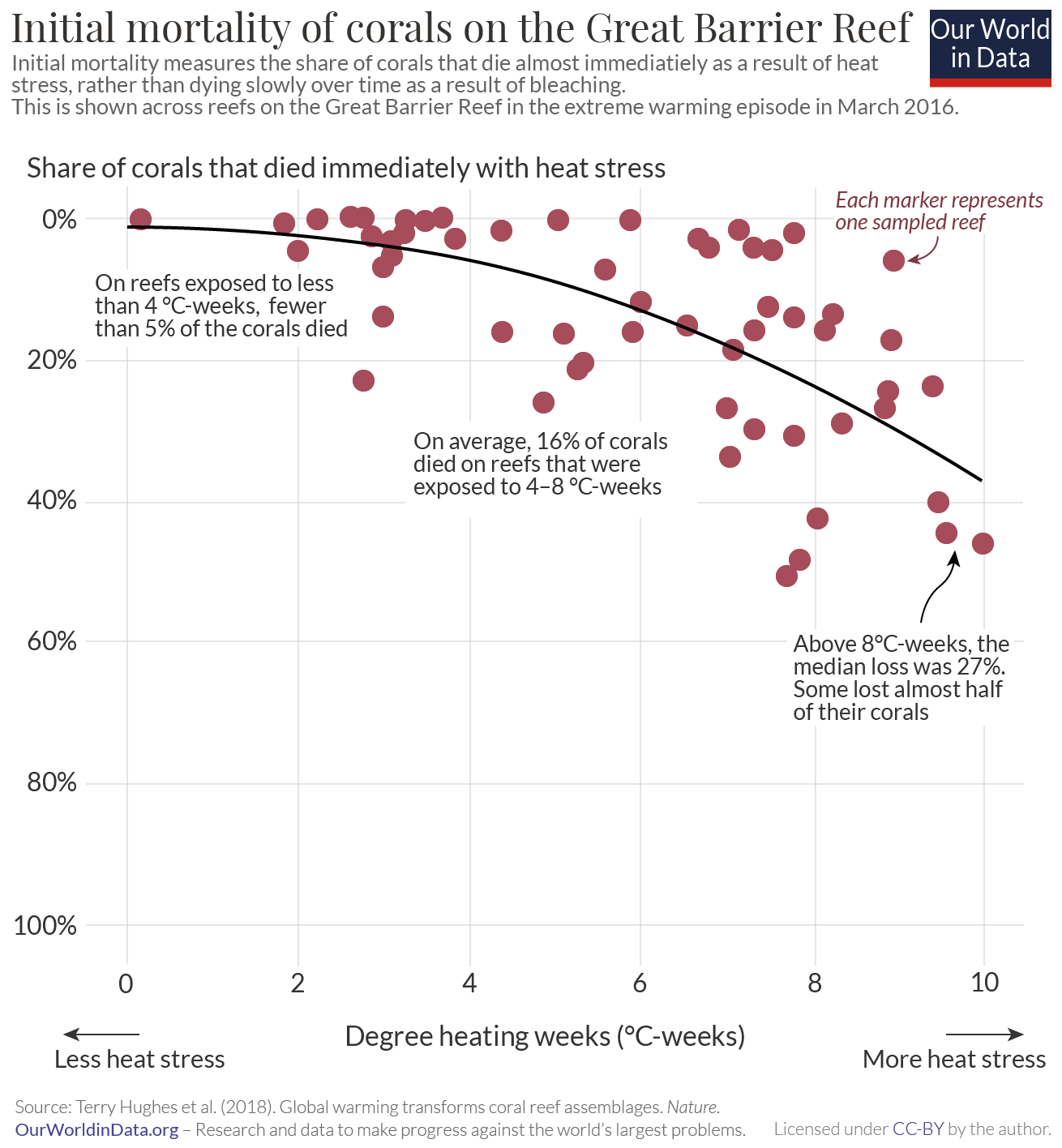
So, some corals die off immediately when they’re exposed to extreme temperatures. These deaths are not driven by bleaching. But, more corals can die from bleaching episodes over the months and years that follow.
On the GBR, over the winter that followed the extreme warming episode in 2016, corals took one of two paths. Some survived and recovered their algae. This means they regained their color. In fact, nearly all of the surviving corals got their color back quickly: less than 1% of the survivors were still bleached after eight months had passed. Other corals did remain bleached and continued to die off over the winter months. They could not recover their algae and the vital energy source that they provided.
In the chart we see the relationship between the reduction in coral cover – the amount of corals that die off – and the extent of coral bleaching. As we might expect, there is a strong relationship between the amount of corals that are bleached and the amount that die off. On reefs with less than 25% bleaching, there was very little loss of coral after eight months. But above this level of bleaching, reefs lost more and more coral with further bleaching.
In the right-hand panel, we also see how the change in coral cover relates to exposure to heat stress. As we might expect, reefs that experienced more heat stress tended to see a larger reduction in coral cover. More heat stress led to more bleaching which led to more long-term coral loss.
But, this response wasn’t linear. There was a threshold beyond which corals died off very quickly. Reefs exposed to less than 3°C-weeks lost almost none of their corals to bleaching. But by 4°C-weeks this dropped off steeply to a decline of 40%. At 8°C-weeks, two-thirds of coral cover was lost. And under very extreme heat stress of 9°C-weeks or more, most of the corals bleached and could not recover.
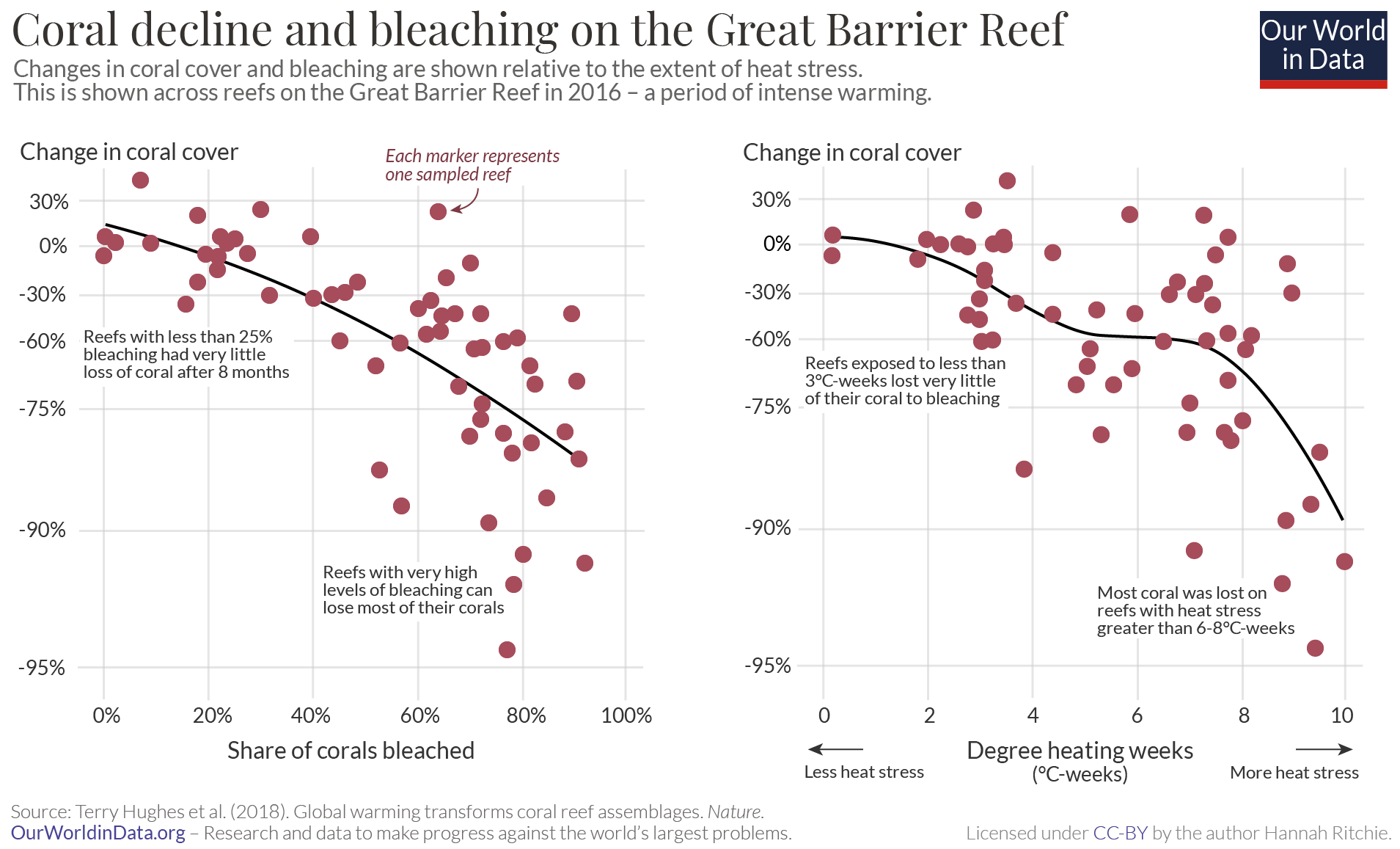
Just like any other animal, corals span a wide range of species. These species have different characteristics, shapes, and grow at different rates. Some grow like platelets; others like branching trees. Some grow rapidly; others much more slowly. This means that reefs can have very different compositions depending on the types of corals that are present.
Coral taxa respond differently to heat exposure. Some are highly intolerant to heat stress and die off immediately, while others can withstand high levels of stress and recover quickly. This creates ‘winners’ and ‘losers’ during bleaching episodes. For example, the tabular staghorn Acropora, Seriatopora hystrix, and Stylophora pistillata are fast-growing branching species that dominate many reefs in the Indo-Pacific region. But, they’re also very vulnerable to bleaching episodes and experience a high mortality rate. On the GBR, these species declined by more than 75% during the 2016 bleaching event.
The fact that there are winners and losers means that it’s not just the amount of coral on a reef that changes during extreme temperatures, but also its composition. It becomes more dominant in the resilient species and loses all of the vulnerable ones. Researchers can measure changes in reef composition using a metric called the nMDS score. A higher score means a larger change in the make-up of a reef ecosystem.
In the chart we see the shift in composition across the sampled Great Barrier reefs in 2016. This is measured relative to the amount of heat stress that each reef experienced.
Again, we see that the relationship is not linear. On reefs exposed to less than 6°C-weeks, there was very little change in structure. At lower levels of heat stress, bleaching levels are lower and there is not much differentiation between winners and losers. But above 6°C-weeks there is a dramatic shift in composition as the losing species die off quickly. This leaves only the species that can withstand high levels of heat stress. They become the dominant species, which can completely transform reef ecosystems.
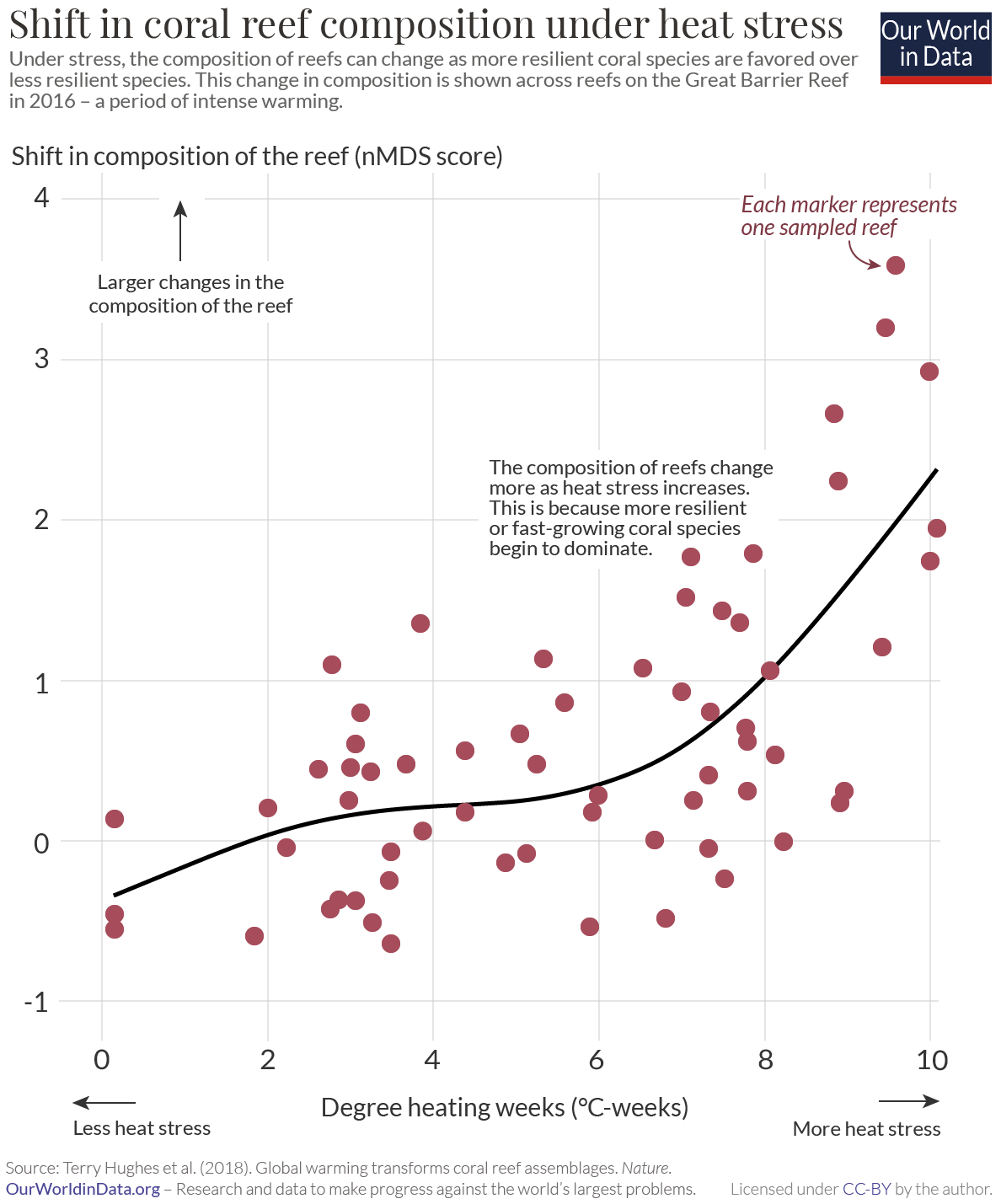
With satellites, surveys and climate modelling we can now track changes in the atmosphere and the oceans at high-resolution. This means scientists can understand when and where we would expect coral bleaching to be a problem in any given season. The US National Oceanic and Atmospheric Administration (NOAA) make forecasts of coral bleaching continuously, and provide alerts when corals are exposed to extreme temperatures.
To make these forecasts we need to make assumptions about what levels of heat stress lead to seriously bleaching, and mortality. But, these recent studies on the Great Barrier Reef suggest the current guidelines on tolerable levels of heat stress might be too low. The NOAA provide guidance that corals experience significant bleaching at 4°C-weeks, and possible mortality at 8°C-weeks.25 Other modelling studies on the resilience of the GBR to climate change assumed that corals only begin to die off when heat stress is over 6°C-weeks.
The experience of the 2016 heatwave on the Great Barrier Reef suggests that these guidelines are too high. Corals can bleach and die at lower levels of stress. As we’ve seen, many corals died immediately at 3 to 4°C-weeks. Many reefs lost more than half of their corals once they reached 4 to 5°C-weeks.
As our oceans continue to warm, this is bad news for the world’s reefs. The loss of many of its corals is almost an inevitability. The biggest thing we can do to protect them is to slow global climate change by reducing our greenhouse gas emissions as quickly as possible.
How do bleaching events vary across the world’s coral reefs?
Globally we have seen an increase in the frequency and intensity of coral bleaching events. This has been driven by increasing temperatures, with warmer waters putting corals under stress.
The frequency of severe bleaching events – where more than 30% of the reef’s corals are bleached – has increased significantly in recent decades. The average time between mass bleaching events has fallen five-fold, from once every 27 years in the 1980s to once every 5.9 years in 2016.
This is a truly global change. Very few corals have managed to escape these impacts completely. Most reefs in the world have experienced bleaching events in the last few decades.
But, there are still distinct differences in the intensity of bleaching across the world’s reefs. All have been affected, but some more than others. In this article, I look at how coral bleaching varies from ocean to ocean, and whether this has changed with warming waters in recent decades.
Before we look at the bleaching trends for specific oceans, there is a larger meta-point about the disproportionate impact on some reefs versus others.
Most of the world’s coral reefs lie in the tropics or subtropics. But there appears to be a marked distinction in the vulnerability of corals across these zones. Reefs that lie closest to the equator have often fared better; those across mid-latitudes experienced worse bleaching. This is despite the fact that corals in both zones have been exposed to similar levels of thermal stress. We see this in the map here which shows the intensity of bleaching across the world’s coral reefs. Purple markers highlight reefs where very little (only one to a few percent) of the corals were bleached. Yellow markers show reefs where most of the corals were affected.
Reefs closer to the equator might be less vulnerable to bleaching because they have adapted to larger swings in temperature over time. Equatorial reefs often experience much more variability in temperature over short timeframes: they see large swings of heating and cooling on a daily basis. This exposure to constant fluctuations might have made them more resilient and adaptable over time.
Reefs at higher latitudes will have less experience of this. When larger changes in sea surface temperature occur, they are less prepared.
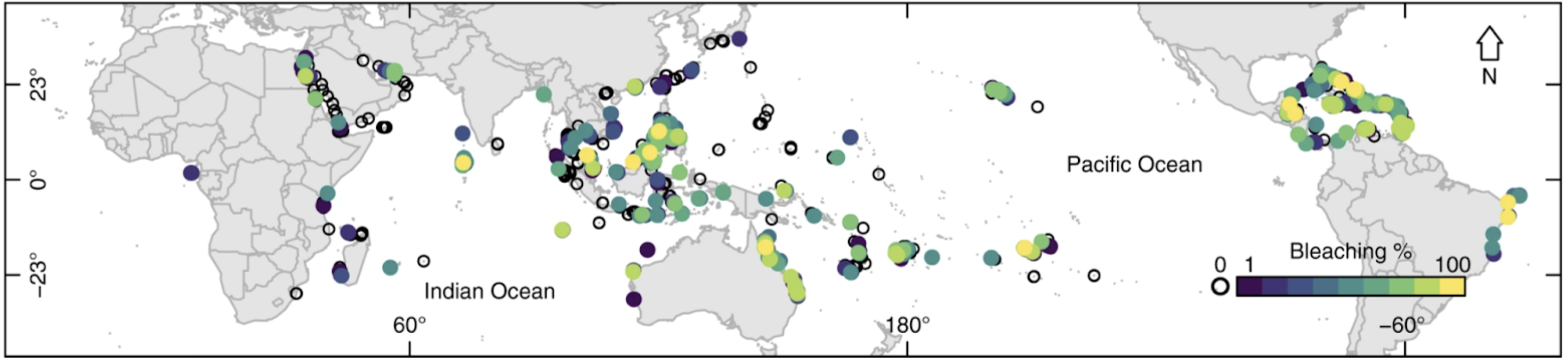
Let’s then take a closer look at how reefs across each of the world’s oceans are affected.
In a study published in Science, Terry Hughes and colleagues tracked the frequency of coral bleaching events across 100 pantropical locations from 1980 to 2016.26 This included all of the key coral hotspots spanning 54 countries, from the West Pacific, to the Atlantic, the Indian Ocean to the Great Barrier Reef in Australasia.
They not only quantified the total number of bleaching events but also their intensity. Bleaching events are differentiated into two categories: moderate bleaching occurs when less than 30% of the corals at a given location are affected; severe bleaching is when 30% or more are. Severe episodes represent events where mass, rather than localized, bleaching has happened.
In the charts here we see the number of moderate and severe bleaching events that occurred in each ocean from 1980 onwards. There are large geographical differences in the timing, intensity, and frequency of these events.
The Western Atlantic warmed earlier than elsewhere, and therefore also saw an earlier uptick in the frequency of bleaching. Its reefs were experiencing regular bleaching much sooner – as early as the 1990s. By 1998 its was seeing an average of four bleaching events per location, compared to 0.4 to 1.6 in other oceans. Over the course of the entire period – from 1980 to 2016 – the Western Atlantic has seen the highest number of bleaching events, averaging ten events per location. This is two to three times higher than other regions.
But it’s not just the number of bleaching events that’s different, but also the intensity. It has also seen the greatest number of severe mass bleaching events.
The Western Atlantic might have been the most exposed to bleaching in the 1980s, but this has also changed a lot over time. In the 1980s bleaching risk was highest in the Western Atlantic, followed by the Pacific, with relatively low risk in the Indian Ocean and Australasia. But this has since flipped.
We see in the charts that the Indian Ocean and Australasia have seen the strongest rise in bleaching over time. In the 1980s, bleaching events were rare. By now it’s rare that there would be a bleaching-free year. This is even true of severe mass bleaching events.
The return times of severe bleaching events – how long following an event that we would expect another – have declined in all regions apart from the Western Atlantic. In contrast to the earlier period, the Western Atlantic managed to escape a major bleaching event between 2010 and 2016. This shortening of return times for many of the world’s corals is obviously bad news for overall reef health: corals can recover from intense bleaching but need enough time between events to do so. For most, this is 10 to 15 years. Getting hit by one bleaching episode after another means corals will struggle to recover.
As we’ve seen, most of the world’s corals are under increasing pressure from bleaching. But they differ in how much pressure and rate that this has changed over time. What might explain these differences?
An important finding from this study was that there doesn’t seem to be a relationship between the average level of warming and the change in bleaching. The corals that had seen the largest rise in bleaching were not necessarily the ones that had encountered the biggest increase in average water temperature. This might seem counter-intuitive since bleaching is driven by warming waters. But it supports the understanding that it’s specific pulses of high temperature – in other words, large changes in the variability – that causes bleaching. It’s about larger extremes in temperature – often over the course of only a few weeks – rather than the background level that matters most.27
This point matters for our understanding of how reefs will be affected by bleaching in the future. It’s tempting to try to make projections based on the expected rise in average sea surface temperatures. This is relatively simple to do. But the relationship to bleaching is a bit more complex. Instead, we need to understand how warming oceans will affect the frequency and intensity of short-term episodes of extreme warming.
An important question is whether reefs will be able to adapt to these extreme temperatures. And if some, but not all of them can, whether it will mean very different coral ecosystems from what we have today. In our follow-up article we look at how adaptable our corals might be to a warming ocean.
Can coral reefs adapt to a warming ocean?
As our oceans get warmer with climate change, the world’s coral reefs are experiencing more frequent and more intense bleaching episodes. This is when they expel their algal symbionts – which are their primary source – due to environmental stress. Some corals die immediately when exposed to extreme temperatures. Others become bleached then either recover or slowly die over the coming months. As the time between successive bleaching events get shorter and shorter, corals do not have the time they need to recover.
Under current warming trends, the future for many of the world’s corals looks bleak.
Some have suggested that there might be a silver lining. Perhaps coral reefs are more resilient than we give them credit for, and can adapt to a warming ocean. The broader question of whether corals can adapt touches on two related but distinct questions.
First, the question of whether an individual coral becomes more resilient to bleaching over time. If a coral experiences coral bleaching, are they more resilient to future events? Effectively, is there a protective effect of past bleaching?
Second, the question of whether coral reef systems can adapt. If particular coral species are more resilient than others, perhaps the reef as a whole can continue to thrive by ‘selecting’ for the species that can survive.
In this article we take a look at the evidence for whether we see this adaptation happening, and what the future of the world’s corals might look like.
If a coral experiences and recovers from an intense bleaching episode, will they be protected from another event years later?
There are a couple of ways in which organisms can acclimatize or adapt to different conditions. Organisms can often adjust to new environments – such as a change in temperature, pH, moisture level or altitude – to make sure they can survive across a range of conditions.28
We see many examples of this in nature. Sheep, for example, grow thicker coats in colder climates then shed them in the Spring or Summer. We even see examples in humans. When we climb a mountain our bodies produce more haemoglobin – the protein in red blood cells – which transport oxygen around the body. This helps us to adapt to higher altitudes. When we move to a hot climate our bodies adjust by sweating at a lower core body temperature while also reducing the amount of salt in our sweat.29
In corals, there are a couple of ways that this might work.30 Some involve changes in the coral host, and some in the symbiont.
First, the coral hosts can release higher levels of specific genes involved in stress-resistant traits. By releasing heat shock proteins and antioxidants, they can respond efficiently to pulses in warming and cooling. One study, published in Science, took the species Acropora hyacinthus – an important coral in the Pacific that tends to bleach easily – and transplanted it to an environment with very frequent swings in temperature.31 The corals acquired heat resistance in their new environment. This resistance that comes with being in a rapidly shifting environment mirrors the heat tolerance we see in corals elsewhere: corals close to the equator tend to experience much less bleaching than those at mid-latitudes; this is because they experience much larger swings in temperature on a day-to-day basis. They’ve adapted resilience to warming and cooling pulses.
Second, acclimatization traits could be passed onto offspring. When corals reproduce, most of the inheritance of key traits is based on genetic factors. But experiments on species such as Acropora, Goniastrea, Platygyra and Porites, suggest that some acclimatization from generation-to-generation could influence the tolerance of offspring.32 However, the problem with this type of acclimatization is always the timescales involved: generational cycles tend to be 4 to 8 years long.33 This might not be able to keep pace with warming.
The third involves the selection of particularly heat-resistant symbionts. There are large differences in how well different types of Symbiodinium can repair photosynthetic damage – the cause of heat stress and bleaching.34 Some, but not all, corals can become more resistant to warming by selecting for these heat-tolerant species.35 One symbiodinium that is very tolerant to changes in temperature is the so-called D1 (‘Symbiodinium trenchi’).36 We find that corals accumulate much more D1 symbionts in waters that experience higher levels of warming. These are often much more resistant to bleaching.
There is mixed evidence on how well these three processes work to protect reefs in practice. Some results suggest that the threshold for coral bleaching has increased over the course of decades, or even years. Corals species that bleached heavily across the world’s reefs in the 1990s seem to show more resistance to bleaching since the millennium. There’s evidence of this in multiple locations including the Great Barrier Reef; the Maldives; Moorea in the French Polynesia; and Southeast Asia.37
In contrast, more recent studies on the Great Barrier Reef have found no evidence of a protective effect of past bleaching.38 The reefs that experienced the most bleaching in the 1998 and 2002 warming events did not fare any better than others in the 2016 event. They bleached just as much. This would suggest that previous exposure did not improve their resilience to subsequent bleaching events.
It’s important to note is that the 2016 warming event was a particularly extreme one. It was the largest mass bleaching event on record in Australasia.39
This might point towards an explanation for why we have conflicting results – that some studies show that previous bleaching provides some adaptive protection while others do not. Perhaps reefs can and do adapt to previous heat stress, but only up to a certain point. There may be a limit to what they can adapt to. Their adaptive success might show in more moderate bleaching episodes but when extreme events – like the Great Barrier Reef bleaching in 2016 – arrive, they are pushed beyond their limits.
How well corals can acclimatize or adapt to climate change will therefore depend on the frequency and intensity of these very extreme bleaching episodes.
There might be another way that reefs can continue to survive, or even thrive, in a warming ocean: by ‘selecting’ for the most resilient coral species. In this case we’re not talking about the adaptability of individual corals but the adaptability of the reef system as a whole.
Let’s say we have a reef that is built from two coral species: Coral A is very intolerant to warming events and dies off quickly; in contrast, Coral B is hardy and bounces back quickly after 1°C or 2°C of warming. The prospects for Coral A over the next century don’t look good: after several mass bleaching events most of it is gone. But this doesn’t necessarily mean that our reef is gone. Coral B is still going strong and soon takes the place of the disappearing Coral A. In the end our reef system looks very different to how it started. But it’s there nonetheless.
How does this play out in the real world? Do we really see such large differences in the tolerance of different coral species?
We already know that different species can tolerate very different environmental conditions. The temperature, pH or salinity of the world’s oceans are not the same everywhere: corals in the Red Sea can withstand different conditions to corals on the Great Barrier Reef or the Arabian Sea.
Corals in the Persian Gulf already exist in waters that reach 34 to 35°C in the summer.40 That’s already warmer than what most of the world’s corals would have to survive by the end of the century with climate change. So it’s not impossible for corals to withstand these temperatures. The caveat is that these corals probably adapted to these temperatures over the course of thousands of years, rather than the decades that today’s corals have to adapt to climate change.
Rather than comparing corals in different environments we should compare the bleaching or mortality thresholds of species within their current environments. Even there we see large differences in response from species to species.
Coral taxa show large differences in their sensitivity to bleaching
In a study in Moorea in the French Polynesia, researchers looked at how different species of coral were affected in a mass bleaching event in 2007.41 They studied four common corals – Acropora, Montipora, Pocillopra and Porites – and looked at how badly each of them were bleached. This ‘bleaching susceptibility’ was measured as the percentage of corals that were bleached weighted by how severe the bleaching was. The results are shown in the chart.
Acropora – also known as ‘elkhorn coral’ because it has branches that look like antlers – was the most intolerant to heat stress. Bleaching susceptibility was more than 50% in colonies of all sizes (from less than 10 centimeters to more than 50 centimeters). Montipora experienced moderate bleaching. The susceptibility of Pocillopora was very different: small colonies of these species were very tolerant to heat stress, but the largest ones experienced a lot of bleaching. Finally, at the bottom we have Porites, a stony coral that tends to form small finger-like structures. It was very resistant to heat stress – almost none of its corals experienced bleaching.
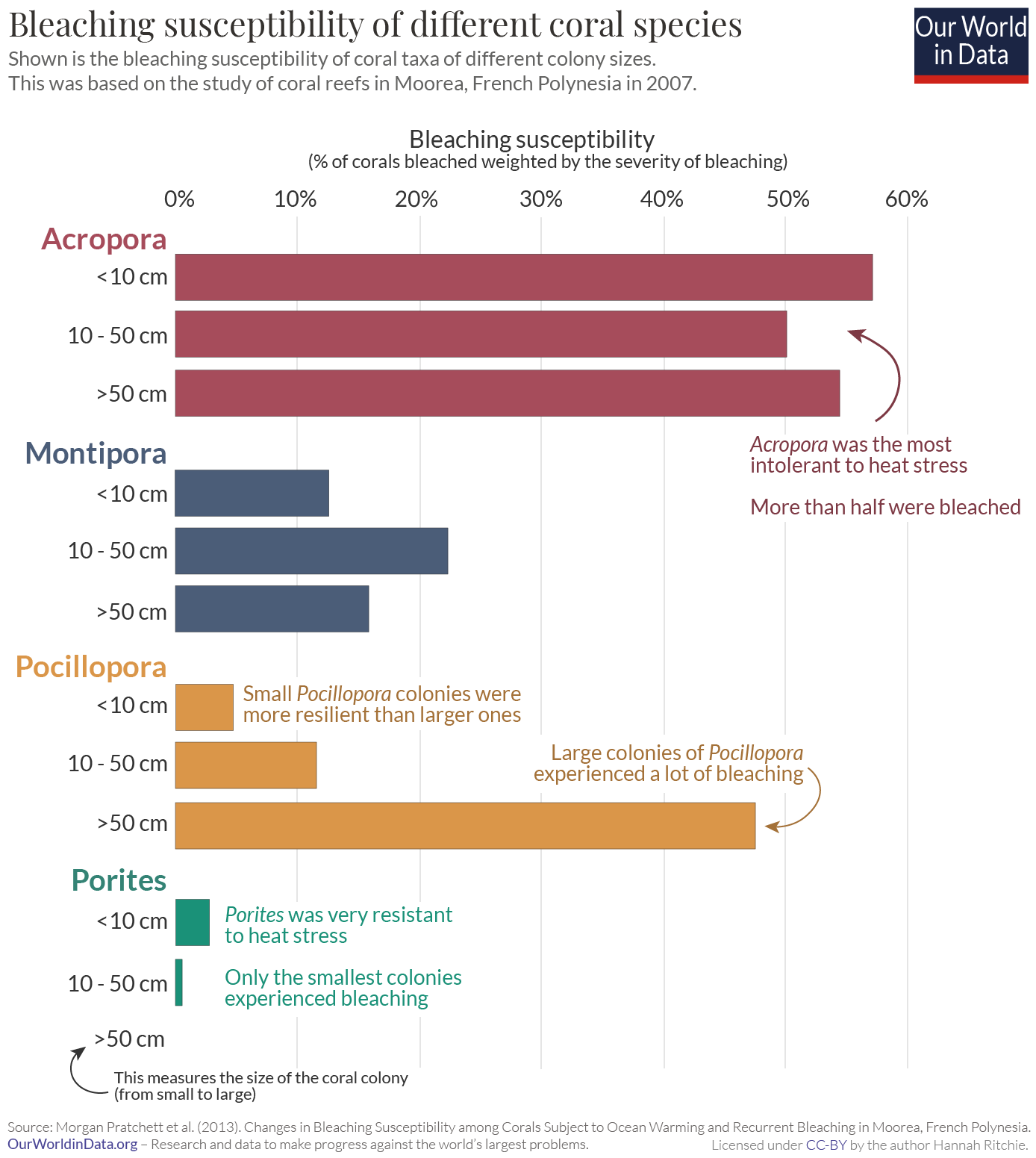
This variability in response to heat stress is not unique to these particular coral taxa. A number of studies have shown the same across a range of coral taxa and reefs across the world.42 One study looked at the bleaching susceptibility of coral taxa in Florida Keys over the course of eight years.43 In the chart we see how the probability of corals bleaching changed with increasing heat stress. Heat stress is measured using a metric called ‘degree heating weeks’ – more degree heating weeks equals more heat stress.
As we’d expect, the probability of bleaching increases with increasing heat stress. This is true of all coral taxa. But there are large differences in the sensitivity of each. As we saw from our study in the French Polynesia, Acropora is highly susceptible to bleaching and comes out on top. But what’s interesting is that this is only true when heat stress reaches a certain threshold – around four degree heating weeks. Other taxa – such as Siderastrea siderea and Stephanocoenia intersepta – experience small amounts of bleaching even at very low levels of heat stress. The difference is that these corals are much less responsive to even more heat stress.
This means that not only do different corals respond differently to warmer temperatures, the magnitude of these differences really depends on how extreme the warming event is and the amount of stress they are put under.
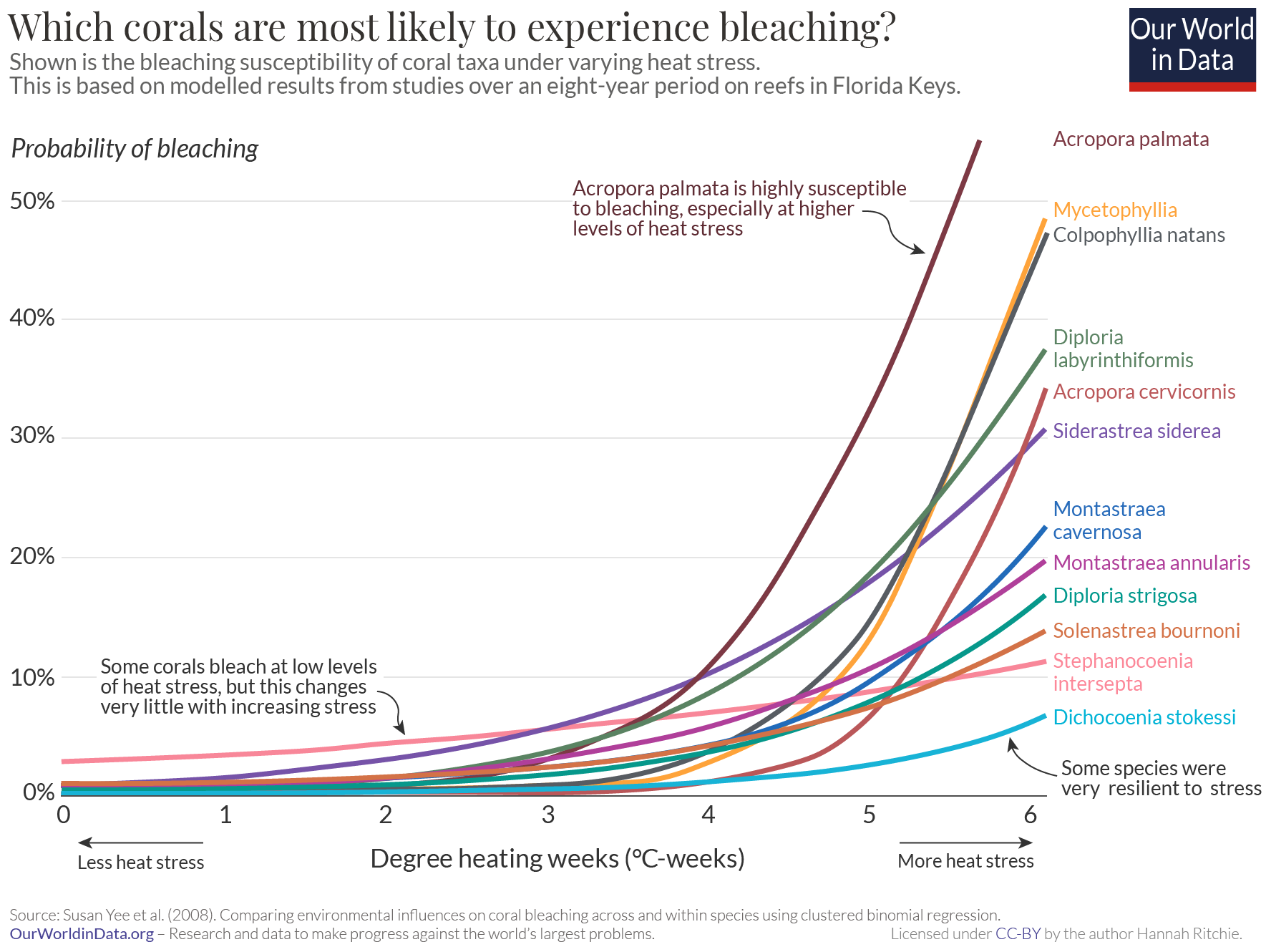
Changes in reefs will also reflect differences in how quickly corals recover and grow
If some corals bleach – and possibly die off – much more easily than other species, it seems likely that reefs will begin to be dominated by the most resilient ones. This seems likely. But sensitivity to bleaching is just one part of the story.
We also need to consider how quickly corals can recover and grow. If corals that bleach easily then bounce back quickly, they might be able to maintain their spot on the reef. Conversely, if more resilient corals that experience only moderate bleaching take 10 to 20 years to recover and grow back slowly, they could lose theirs.
Researchers have looked at this dynamic between some specific corals in detail. The two corals which dominate many reefs in the Indo-Pacific region – which includes corals off the coasts of Southeast Asia and the Great Barrier Reef – are Acropora palmata and Porites. These corals could not be more different. As we’ve already seen, Acropora bleaches easily when exposed to high levels of heat stress. Porites is much more resilient. But their growth rates and recovery times are also very different: Acropora grows quickly; Porites grows slowly.
This means infrequent but severe disturbances tend to favor Acropora because it can grow back quickly. But moderate, frequent events tend to favor Porites which is much less-affected by moderate warming. Models that look at the dynamics of these two corals on a reef system suggest that Acropora continues to dominate as long as the interval between bleaching events is more than two years.44 Since it grows quickly, two years gives it sufficient time to recover and grow. If the interval between events is less than two years then Porites starts to dominate because even the fast-growing Acropora cannot recover quickly enough.
Researchers therefore expect susceptible corals like Acropora to decline in abundance as a result of increased warming. But depending on the frequency of bleaching events they may not decline by as much as their response to heat stress would suggest.45
As we’ve seen, corals can adapt to changing environments in multiple ways. The corals themselves can adapt and acclimatize to changing temperatures through genetic changes in the host, or changes in the selection of heat-resistant symbionts. But reefs as a whole can also change and adapt, with more resilient species becoming more dominant while others die away.
It’s unlikely that the coral reefs of the future will look like they do today. Reef assemblages will be different. Just how different, and how much coral cover we will lose completely will depend on the intensity and frequency of extreme bleaching events.
The research suggests that corals themselves can adapt to changes – but only up to a certain point. More and more extreme events will push beyond these limits. And the assemblages of coral reefs will depend on how frequent bleaching episodes are – fast-growing corals have a chance of survival as long as bleaching events don’t happen every year or two.
Human emissions are driving climate change. The intensity of coral bleaching depends on how much greenhouse gases we emit. The future of the world’s coral reefs rests on the decisions we make to tackle climate change today.
What impact will ocean acidification have on coral reefs?
When we think of the impacts of carbon dioxide (CO2) emissions we tend to focus on its impact on climate change. But for marine organisms, these emissions pose a double threat. CO2 emissions could risk the future of marine life, including coral reefs through ocean acidification.
Before we look at the potential impact of ocean acidification on the world’s corals, we should first understand what it is, and why it happens.
How much CO2 exists in the atmosphere is determined by the balance of how much is emitted, and how much is absorbed by land and ocean ‘sinks’. If we emit more than can be absorbed by forests, other vegetation and the ocean, then the atmospheric concentration increases. The Global Carbon Project shows this nicely in the graphic.46
What this means is that the ocean absorbs a lot of CO2. As we can see from the chart – the amount of absorbed CO2 has been increasing over time as a result of increased emissions from fossil fuels. So it’s not just the atmospheric concentration that has been increasing, but also the concentration in the ocean.
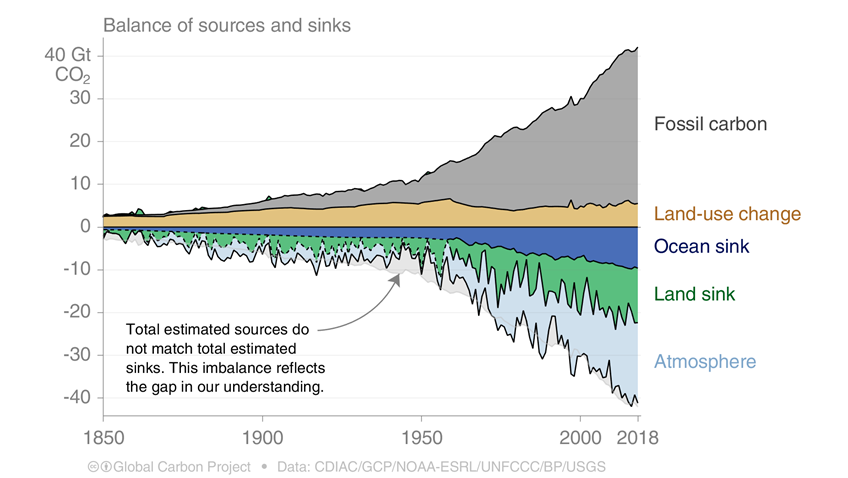
Now, on to chemistry. When CO2 is absorbed in water, it reacts to form other substances. When we combine CO2 and water (H2O) we get carbonic acid. (H2CO3). This reaction occurs:
CO2 + H2O → H2CO3
H2CO3 can then lose its hydrogen atoms to form bicarbonate (HCO3) then carbonate (CO3) ions.
H2CO3 → HCO3 + H+
HCO3 → CO3 + H+
You might remember that the acidity of water – its pH – is driven by the concentration of hydrogen (H+) atoms. More H+ atoms makes water more acidic. So we can see that through these reactions, our seawater becomes increasingly acidic. This chain of reactions was started by adding CO2 to the water.
But that’s just part of the story. The pH of water does decrease (being more acidic) when we add CO2, but not quite as much as we might expect. This is because water tries to ‘buffer’ against this acidity. It creates reactions to work against this process. Some of the CO2 that’s added to water also reacts with carbonate ions (CO3) to form bicarbonate ions:
CO2 + CO3 + H2O → 2HCO3
Overall, there are two outcomes of adding CO2 to water. First, the pH decreases a bit and our water becomes more acidic. Second, some carbonate (CO3) ions are consumed to buffer against this process.
Why does this matter? Why are carbonate ions so important? Corals and other marine life build their shells and exoskeletons using the mineral calcium carbonate (CaCO3). To build them, they need carbonate ions in the water. Clearly, as carbonate reacts with CO2, we end up with less and less in the water. Less carbonate for marine life to form their shells.
Whether carbonate crystals form depends on what we call the ‘saturation state’ (𝛀) of seawater. The saturation state is the ratio between the amount of carbonate ions in seawater, and the ‘saturation’ amount for carbonate ions in the water. The ‘saturation’ concentration depends on the temperature, salinity, and depth of the water.
Ω = [CO3 seawater] / [CO3 saturation]
If there is enough carbonate (CO3) in the seawater, then Ω is greater than 1 (it’s supersaturated) and carbonate crystals can form. If there is not enough CO3, then Ω is less than 1 (it’s undersaturated) and so they can’t form.
We can see how this will affect corals and other marine organisms: when ocean acidification happens and the amount of carbonate ions in seawater decreases, the value of Ω decreases. Without continued acidification, Ω could fall below one. Corals then risk the dissolution of their current skeletons and struggle to form new ones.
When the erosion rate of organisms is higher than the rate that they can build new skeletons, they slowly disappear.
This leads to the question of how much ocean acidification we might expect to see in the coming century. Is it large enough to shift seawater from its ‘supersaturated’ state where corals and other organisms can form? Or will reefs struggle to survive and grow?
There are two elements of this question. First, how do we expect our emissions of CO2 to affect the chemistry of seawater? Second, how will corals respond to these changing conditions?
Scientists can try to test future acidification in controlled experiments. They can expose corals to waters with different concentrations of dissolved CO2 – mimicking the process of human emissions – and monitor how they respond. Many experiments have tried to look at the response of corals to this acidification.
It’s actually very difficult to say what the future of coral reefs look like for the coming century. This is because most of the experiments have exposed corals to CO2 concentrations that are much higher than we expect to see in the real world. As we cover in a related article, Hughes et al. (2017) reviewed 336 experiments across 137 studies and found none that assessed the impacts of concentrations in the range of 400 – 500 ppm, the concentrations that we’d expect coral reefs to experience in the coming century.48 The lowest concentrations started at 500 ppm: one-third of the experiments ranged from 500 to 750 ppm. Another third ranged from 750 to 1000 ppm, and the remaining third were over 1,000 ppm.
A meta-analysis of the sensitivity of corals to acidification found that calcification declined by around 15% when Ω fell by one unit.49 What would it take for Ω to fall by one unit? Atmospheric concentrations of CO2 would have to almost double from around 400 to 800 ppm. Even the worst (and unrealistically higher) scenarios of CO2 emissions barely reach 800 ppm by the end of the century. It’s incredibly unlikely that we reach this point.
At lower levels, these studies have suggested that there is a ‘threshold’ concentration of around 560 ppm. The understanding is that below 560 ppm, most corals are still in a ‘supersaturated’ state. They continue to grow as normal. But above 560 ppm, their rate of calcification begins to decline. This concentration of 560 ppm is getting closer to the concentrations of CO2 that we might begin to see by the end of the century. But, due to the lack of experiments that have focused on coral responses in the range of 400 to 500 ppm, we still don’t have a good understanding of what the impact on corals might be over the coming decades.
The world’s coral reefs face a double-threat from carbon dioxide emissions. They can bleach or die from warming oceans but also by ocean acidification; the dissolution of CO2 in the oceans can make it increasingly difficult for corals to build their carbonate exoskeletons.
To understand the future prospects of the world’s corals we need to know how they might respond to changes in temperature and CO2 concentrations. This needs to be in line with realistic projections of what we might expect from emissions in the coming decades or century.
We’ve set a global climate target of keeping average temperature rise to “well below 2°C” and to “pursue efforts to limit the temperature increase to 1.5 °C”. We’re currently not on track to achieve either of these targets, and are instead on course for an average warming of around 3°C above pre-industrial temperatures by the end of the century. But, we’re now thankfully well below the extreme ‘business-as-usual’ pathways that would take us to 6°C, 7°C, 8°C warming and CO2 concentrations of 6000 to 1000 ppm.
It’s important to note that the 1.5 °C and 2 °C targets are less relevant for coral reefs. The oceans experience different changes in temperature. The average tropical sea surface temperature increased by 0.57 °C between 1880 and 2015. That’s lower than the 0.88 °C increase in global average temperature for the same period. There is also a lot of spatial and temporal variation across the oceans: corals are particularly vulnerable to spikes in summer water temperatures, and this can vary a lot from location to location. Due to this heterogeneity, there is no single ‘safe’ level of warming for coral reefs.
If the world was to follow the lowest emissions scenario (RCP2.6) we’d expect to see a warming of 0.3°C to 0.68 °C between different reefs. That’s an increase similar to the warming we’ve already seen over the past century. What would be the impact on the world’s corals?
We don’t really know. The problem is that most experiments which look at the temperature and acidification effects on corals have focused on temperatures and CO2 concentrations that are much higher than this. In a paper published in Nature, Terry Hughes and colleagues looked at the range of experimental studies that had been done on coral responses to climate change.50 This involved 336 experiments across 137 studies. These experiments expose corals to one or more treatments of elevated water temperatures for periods ranging from a single day to a year or longer. Or in the case of ocean acidification, expose them to waters with varying concentrations of dissolved CO2.
The chart shows the distribution of experimental conditions in the studies. The height of each bar represents how often a given temperature or CO2 concentration had been assessed.
We see that almost none of the studies looked at effects of up to 1°C or 2°C warming that we would expect over the next century. The median change across the experiments was an increase of 4°C (almost 5 °C above pre-industrial temperatures).
In fact, no study had done experiments on the biological response of corals to temperature increases in the 0.30–0.68 °C range that we would expect under the RCP2.6 scenario of limiting warming to “well below 2°C”.
The same is true for experiments on ocean acidification. Since pre-industrial times, the average pH of the ocean surface waters has declined by about 0.1 units, from pH 8.21 to 8.1.51 Some of the research on ocean acidification has painted a bleak picture for the world’s coral: studies have suggested that the pH of ocean waters will fall by another 0.3 to 0.4 units, and reefs across the subtropics will dissolve. This will confine the optimal conditions for coral calcification to a narrower band across the equator.
But, these studies are based on very high emissions scenarios (such as RCP8.5) where CO2 concentrations reach 800 ppm. This is not what we should expect. If we were to stabilise atmospheric concentrations of CO2 at 450ppm, the pH of the world’s oceans would remain at around 8.1 in most tropical waters – this would keep them in an optimal state for coral calcification.
As we see in the chart, most experiments have been conducted at very high CO2 concentrations. Most experiments were designed to match conditions of unconstrained greenhouse gas emissions.
Hughes et al. (2017) reviewed 336 experiments across 137 studies and found none that assessed the impacts of 400 – 500 ppm, the concentrations that we’d expect coral reefs to experience in the coming century. The lowest concentrations started at 500 ppm: one-third of experiments ranged from 500 to 750 ppm. Another third ranged from 750 to 1000 ppm; and the remaining third were over 1,000 ppm.
Not only are most of these implausibly high, they also simulate conditions in a world that would have killed off most of the world’s corals regardless. A world with CO2 concentrations of over 1,000 ppm would be at least 6 °C warmer than today. The corals would have died from severe warming already. They wouldn’t be around to experience these levels of ocean acidification.
What this review highlights is the massive gap in our understanding of what corals will face under realistic warming and acidification conditions over the next century. We need to adjust the range of temperature and pH conditions that we test corals under. And importantly, test what the combined impact of warming and acidification might be under the more realistic range of scenarios of 1°C to 2°C of warming.
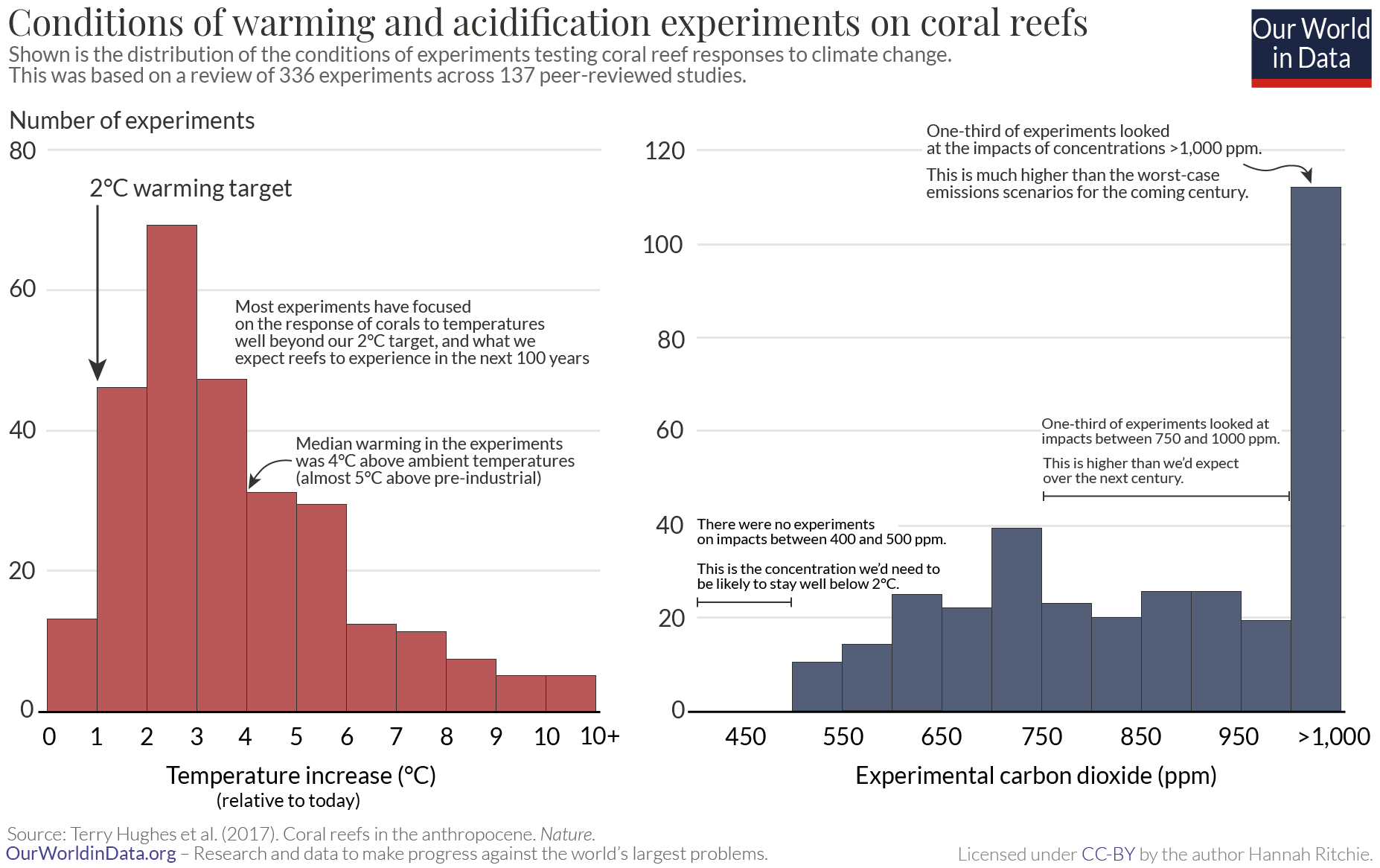